Ammonia
Overview
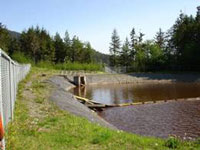
Ammonia is a common cause of fish kills. However, the most common problems associated with ammonia relate to elevated concentrations affecting fish growth, gill condition, organ weights and hematocrit (Milne et al. 2000). Exposure duration and frequency strongly influence the severity of effects (Milne et al. 2000).
Ammonia in sediments typically results from bacterial decomposition of organic matter that accumulates in sediment. Sediment microbiota mineralize organic nitrogen or (less commonly) produce ammonia by dissimilatory nitrate reduction. Ammonia is especially prevalent in anoxic sediments because nitrification (the oxidation of ammonia to nitrite [NO2-] and nitrate [NO3-]) is inhibited. Ammonia generated in sediment may be toxic to benthic or surface water biota (Lapota et al. 2000).
Ammonia also exerts a biochemical oxygen demand on receiving waters (referred to as nitrogenous biological oxygen demand or NBOD). This occurs because dissolved oxygen is consumed as bacteria and other microbes oxidize ammonia into nitrite and nitrate. The resulting dissolved oxygen reductions can decrease species diversity and even cause fish kills.
Additionally, ammonia can lead to heavy plant growth (eutrophication) due to its nutrient properties (see the Nutrients module). Algae and macrophytes take up ammonia, thereby reducing aqueous concentrations.
Checklist of Sources, Site Evidence and Biological Effects
This module addresses ammonia as a proximate stressor with a toxic mode of action. Its nutrient properties are considered in the Nutrients module.
Ammonia should be a candidate cause when human sources and activities, site observations or observed biological effects support portions of the source-to-impairment pathways (see Figure 2).
The checklist below will help you identify key data and information useful for determining whether to include ammonia among your candidate causes. The list is intended to guide you in collecting evidence to support, weaken or eliminate ammonia as a candidate cause.
For more information on specific entries, go to the When to List tab.
Consider listing ammonia as a candidate cause when the following sources and activities, site evidence and biological effects are present:
Sources and Activities
- Impoundments
Figure 2. A simple conceptual diagram illustrating causal pathways, from sources to impairments, related to ammonia. Click on the diagram to view a larger version. - Municipal waste treatment outfalls
- Septic seepage
- Industrial point sources
- Agricultural and urban runoff (fertilizer)
- Manure application
- Concentrated animal feeding operations
- Aquaculture
- Landfill leachate
- Atmospheric sources
- Riparian devegetation
Site Evidence
- Slow-moving or stagnant water
- High density of fish
- Presence of organic waste
- Foul odor
- Presence of organic suspended solids or floc
- Alkaline, anoxic or warm water
- High plant production (e.g., algal blooms)
Biological Effects
- Reduction or absence of ammonia-sensitive species
- Physiological effects (e.g., decreased nitrogen excretion, decreased oxygen binding to hemoglobin)
- Behavioral effects (e.g., loss of equilibrium, hyperexcitability, increased breathing)
- Morphological effects (e.g., proliferation of gill lamellae, reduction of lymphoid tissue in the spleen, lesions in blood vessels, mucus secretion)
- Organismal and population effects (e.g., decreased growth and abundance, mass mortality)
- Temperature: Toxicity of ammonia (as total ammonia) increases as temperature increases (U.S. EPA 1999).
- pH: Ammonia concentration and toxicity increases as pH increases, although less ammonia is required to produce toxic effects at lower pH (IPCS 1986, Wurts 2003).
- Dissolved oxygen: Oxygen is consumed as ammonia is oxidized (nitrification), and low oxygen levels increase ammonia levels by inhibiting nitrification.
- Season: Total ammonia-nitrogen concentration in surface waters tends to be lower during summer than during winter. This is due to uptake by plants and decreased ammonia solubility at higher water temperatures (IPCS 1986).
- Ionic strength: Tolerance to ammonia can increase with an increase in ionic strength or salinity (Sampaio et al. 2002).
- Sediments: Fine sediments tend to generate ammonia due to low oxygen levels and high organic matter.
- Concentrations measured continuously over time at the site are the same as or less than ammonia at sites without observed biological impairment (lack of co-occurrence).
- All life stages of ammonia-sensitive species are present at the site.
When to List
- Sources and Activities that Suggest Listing Ammonia as a Candidate Cause
- Site Evidence that Suggests Listing Ammonia as a Candidate Cause
- Biological Effects that Suggest Listing Ammonia as a Candidate Cause
- Site Evidence that Supports Excluding Ammonia as a Candidate Cause
Sources and Activities that Suggest Listing Ammonia as a Candidate Cause
The amount of ammonia in surface waters is influenced by many human activities in waterbodies and their associated watersheds. The more extensive the relevant sources and activities, the more likely it is that ammonia will reach concentrations that can impair surface waters.
- Impoundments: Impoundment of water may contribute to elevated ammonia concentrations. Lack of turbulence and mixing will decrease volatilization of ammonia, resulting in higher ammonia concentrations downstream. Thermal stratification in impoundments can lead to higher concentrations of ammonia in the hypolimnion which, with bottom-release dams, can result in increased ammonia in downstream waters.
- Municipal waste treatment plants: Municipal waste treatment plants and public-owned treatment works (POTWs) process domestic waste and are operated to comply with permit limits designed to protect receiving waterbodies from excess inputs of pollutants such as ammonia (Constable et al. 2003). However, during storms, excess flow may be diverted into combined sewer overflows (CSOs) that deposit untreated municipal waste directly into streams and lakes. Treatment plant failures also may result in high ammonia releases to streams (see Figure 3).
Figure 3. Discharge from a wastewater treatment plant.
Photo courtesy of USGS Toxic Substances Hydrology Program Photo Gallery - Septic seepage and failed package plants: Seepage from failed septic tanks or their leach fields, and discharges from poorly–functioning package sewage treatment plants may contribute significant amounts of ammonia to streams and lakes.
- Industrial point source: Certain industries release ammonia as a byproduct in their waste stream. These end-of-pipe discharges are regulated via permit limits to protect receiving waterbodies, but where the original system design is inadequate or problems in operation lead to inadequately treated discharges, increased ammonia concentrations result. This is especially an issue for industries that produce ammonia, aminated organic matter, or other nitrogenous wastes such as food processing (e.g., poultry, livestock, or seafood), pharmaceutical manufacturing (e.g., fermentation processes), paper mills, and flue gas treatment in coal-fired power plants.
- Agricultural and urban runoff: Runoff and leachate from agricultural, recreational (e.g., golf courses) or residential fertilizer use can directly increase the amount of ammonia in surface water (see Figure 4).
Figure 4. Agricultural runoff.
Photo courtesy of NOAA Ocean Service Education - Manure application: Manure containing ammonia and other nitrogenous compounds often is spread on fields and then washed into streams and other water bodies by rain or snow melt. Grazing livestock spread urine and manure on pastures and, where they have access to streams, they apply urine and manure directly to water.
- Concentrated animal feeding operations: Runoff from feedlots and other concentrated animal feeding operations can contain high levels of ammonia and other nitrogenous compounds.
- Aquaculture: Drainage from fish and shrimp farms is high in ammonia if not properly treated.
- Landfills: Leachate from landfills may contain high ammonia concentrations (Mancl and Veenhuizen 1991).
- Atmospheric sources: These sources include ammonia originating from agricultural practices and nitrogen oxide emissions from automobiles and industry (NOAA 2000). These are regionally important sources, but are seldom indicative of particular impairments.
- Riparian devegetation: The removal of vegetation from the banks of surface waters increases surface water runoff and water temperature and decreases woody debris input. Increased surface water runoff may increase the amount of ammonia directly entering the waterbody. Increased water temperature enhances the toxicity of ammonia. Reduced turbulence from less woody debris may decrease volatilization and oxygenation.
- High plant production: High algal or plant production can decrease ammonia by assimilation, increase ammonia by nitrogen fixation, or increase pH toxicity by CO2 uptake, resulting in a shift to more unionized ammonia.
Site Evidence that Suggests Listing Ammonia as a Candidate Cause
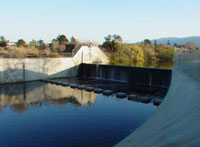
Courtesy of USGS
Slow-moving water: Slow-moving or stagnant water (see Figure 5) may have high ammonia concentrations because of lack of turbulence and volatilization and greater accumulation of metabolic waste and decomposition products—including ammonia (WHO 1986).
High density of fish: Reduced stream flow may concentrate fish into pools or other refugia, concentrating waste excretion and elevating ammonia concentrations.
Presence of organic wastes: Organic wastes are the remains of any once-living organism or their excrement. Excrement and sewage are high in ammonia, so signs of such material in a waterbody may suggest ammonia as a candidate cause. In contrast, plant material is typically low in nitrogen, and associated decomposers may take up ammonia and reduce its aqueous concentration. Identifying the type of organic waste present in a waterbody will aid in identifying candidate causes. Excessive organic wastes in water may result in a grayish cast with visible sludge deposits in depositional areas.
Foul odor: Ammonia as a gas has a characteristically pungent odor (think of window and floor cleaners). Ammonia concentrations in streams are rarely high enough to exhibit this odor, but water that has a foul, septic or organic-waste smell may have relatively high concentrations of ammonia.
Suspended solids: Suspended solids from wastewater effluents or runoff can contain high ammonia or act as catalysts for bacterial growth promoting accumulation of ammonia. Identifying the type of suspended material present is important in identifying candidate causes.
Alkaline, anoxic or warm water: Water characteristics that promote ammonia formation (e.g., anoxia) or increase toxicity (e.g., high pH and temperature) are signs that ammonia may be a cause.
Biological Effects that Suggest Listing Ammonia as a Candidate Cause
Unionized ammonia is very toxic to aquatic animals, particularly fish, because it can readily diffuse across gill membranes (Sampaio et al. 2002). Ionized ammonia does not pass as easily through gill membranes, so it is appreciably less toxic than the unionized form (Camargo and Alonso 2006). In most fish, ammonia is excreted by passive diffusion of ammonia across the gills according to its partial pressure gradient (Wilson et al. 1998). Disruption of this gradient causes internal ammonia concentrations to increase, affecting internal organs, nervous system function, and respiration.
Reduction in ammonia-sensitive species and processes: Salmonids tend to be particularly sensitive in acute exposures associated with episodic sources. However, sensitivities to chronic exposures are less taxonomically consistent. In particular, the most sensitive fish genus used to derive the chronic water quality criterion is the sunfish (Lepomis spp.) (U.S. EPA 1999).
Early life stages of fish are more sensitive than juveniles or adults. Hence, effects are more likely to occur during seasons when early life stages are present.
Invertebrates, particularly arthropods, are generally less susceptible than fish. Hence, observations that fish are more affected than invertebrates are consistent with ammonia as a cause. However, recent information suggests that certain freshwater mussels (Unionidae)—particularly the glochidia and juvenile stages—are very sensitive to ammonia (Newton and Bartsch 2006).
Unionized ammonia can cause toxicity to Nitrosomonas and Nitrobacter bacteria, inhibiting the nitrification process. This inhibition can result in increased ammonia accumulation in the aquatic environment, intensifying the level of toxicity to bacteria and aquatic animals (Carmargo and Alonso 2006).
Physiological, morphological and behavioral effects: Specific biotic effects suggesting that you consider ammonia as a candidate cause are described in Table 1. Please note, however, that observation of these effects does not confirm a causal relationship. In some cases the same observed effect could be caused by other stressors or multiple agents. If you suspect ammonia as the cause of observed biological impairments, then also consider pH, temperature, and low dissolved oxygen—stressors often associated with and contributing to ammonia concentrations.
Effects | Reference |
---|---|
Decreased respiratory function causing hyperventilation | Lease et al. 2003, Twitchen and Eddy 1994, IPCS 1986 |
Impairment of nerve function; peripheral and central nervous system effects causing hyperexcitability | Sampaio et al. 2002, Twitchen and Eddy 1994, IPCS 1986 |
Convulsions | Twitchen and Eddy 1994, IPCS 1986 |
Coma | Twitchen and Eddy 1994, IPCS 1986 |
Damage to gill epithelia causing asphyxiation | Lang et al. 1987 |
Proliferation of gill tissue | Lang et al. 1987 |
Stimulation of glycolysis and suppression of Krebs cycle, causing progressive acidosis and reduction in blood oxygen-carrying capacity | Camargo and Alonso 2007 |
Uncoupling of oxidative phosphorylation, causing inhibition of ATP production and depletion of ATP in the basilar region of the brain | Camargo and Alonso 2007, Sampaio et al. 2002 |
Disruption of blood vessels and osmoregulatory activity, causing stress to the liver and kidneys | Camargo and Alonso 2007, Sampaio et al. 2002, Bosakowski and Wagner 1994 |
Repression of immune system, causing increased susceptibility to bacteria and parasitic diseases | Camargo and Alonso 2007, Sampaio et al. 2002 |
Reduction of Na+ to potentially fatally low levels | Twitchen and Eddy 1994 |
- Reduced growth rate
- Reduced feeding activity
- Reduced fecundity and reproductive success
- Decreased population size
- Increased mortality (e.g., fish kills)
- Increased fish fin erosion
Site Evidence that Supports Excluding Ammonia as a Candidate Cause
Advice on excluding ammonia as a candidate cause is limited to situations in which the physical and chemical characteristics of a site prevent ammonia from logically accounting for the impairment. Thus, a lack of obvious sources and site observations cannot be used to eliminate ammonia as a candidate cause. However, a lack of sources or other evidence may be used to defer consideration of ammonia if other candidate causes are supported.
Sources: Spillways, waterfalls and turbulent flows in streams and rivers naturally volatilize ammonia. Thus, high concentrations of ammonia are physically precluded by consistent volatilization from turbulence. However, ammonia concentrations will be affected if flow changes during the year, and this should be considered. Screening in these situations should be supplemented with measures of ammonia concentrations.
Site observations: We caution against using benchmarks of effects for excluding ammonia from your initial list of candidate causes, because different species have different ammonia tolerances and concentrations are seldom well-characterized. Table 2 provides example toxicity values for selected species with both acute and chronic values from the current Water Quality Criteria for Ammonia (U.S. EPA 1999).
Species | Mean Acute Value (mg N/L) | Mean Chronic Value (mg N/L) |
---|---|---|
Cladoceran, Daphnia magna | 35.76 | 12.3 |
Clam, Musculium transversum | 35.65 | 2.26 |
Fish, Pimephales promelas | 43.55 | 3.09 |
Channel catfish, Ictalurus punctatus | 34.44 | 8.84 |
a LC50 or EC50 means b Means of geometric means of NOECs and LOECs at pH = 8, 25oC |
Ways to Measure
The concentration of ammonia in water is measured as total ammonia and is usually reported in mg/L. The concentration of unionized ammonia (NH3) is derived from well-established formulas (see link to calculator below). When differentiating between unionized and ionized ammonia, it is important to take into account the effects of temperature and pH (Eaton et al. 2005).
- Ammonia probe: A measuring probe is connected to an electronic ion meter that measures and displays the voltage resulting from ammonia, which is then converted to concentration using a standard curve.
- Colorimeter: One or more reagents are added in timed intervals to the sample, such that the intensity of the color produced is proportional to the ammonia that reacts with the reagent. This color absorbance then is measured using a colorimeter or spectrophotometer. Blanks and standards are used to generate a standard curve from which the sample absorbance reading is converted to ammonia concentration.
The fraction of unionized ammonia (NH3) is not directly measured, but instead is calculated using measures of total ammonia, pH, temperature, and ionic strength (measured either in terms of total dissolved solids or conductivity). The American Fisheries Society has developed an ammonia calculator (Table 9 on the referenced web page should be downloaded) for this purpose. Once the required data are entered, the tool will calculate the amount of unionized ammonia in both mg/L and µg/L.
Conceptual Diagrams
About Conceptual Diagrams
Conceptual diagrams are used to describe hypothesized relationships among sources, stressors and biotic responses within aquatic systems.
Simple Conceptual Model Diagram
Sources associated with agriculture, urbanization, industry and aquaculture also can directly increase ammonia inputs to aquatic systems via four main transport pathways (or transport-defined sources): stormwater runoff, leakage or leachate into groundwater sources, atmospheric emissions and deposition, or direct effluent discharges (see Figure 6).
Ammonia is a key component of the nitrogen cycle in streams, where it may be dissolved in the water column or associated with sediments. At high enough concentrations, ammonia can be toxic to aquatic organisms. In general, unionized ammonia (NH3) is the form most toxic to aquatic biota. The relative contribution of unionized versus ionized forms to total ammonia concentrations depends on certain water quality criteria, most notably pH: as pH increases, so does the proportion of ammonia in its unionized form.
Increased ammonia concentrations or fluctuations within streams can result in decreased condition, decreased growth, altered behavior, increased susceptibility to other stressors, increased mortality, and decreased reproductive success in affected biota, and ultimately may alter population and community structure and ecosystem function (Figure 7).
Detailed Conceptual Model Diagram
High concentrations of ammonia in aquatic systems can have lethal and sub-lethal effects on aquatic organisms, potentially changing community structure and ecosystem function. This conceptual diagram (Figure 8) illustrates linkages between ammonia-related stressors (middle of diagram), the human activities and sources that can increase those stressors (top of diagram), and the biological responses that can result (bottom of diagram).
In some cases, additional steps leading from sources to stressors, modes of action leading from stressors to responses, and other modifying factors also are shown. This narrative generally follows the diagram top to bottom, left to right.
Linking Sources and Activities to Proximate Stressors
Channel alteration can increase ammonia concentrations in several ways. Alterations that reduce channel complexity (e.g., piping or channelizing streams) can result in decreased nitrogen uptake. Impoundment of stream channels can result in decreased ammonia volatilization upstream of impoundments, due to decreased water velocities (and increased water depths). Reduced water flows downstream of impoundments (or in streams affected by water withdrawals) can limit habitat availability, crowding biota and concentrating ammonia-rich waste products.
Many human activities and land uses also decrease riparian and watershed vegetation, which may decrease the amount of nitrogen taken up by terrestrial plants, and further reduce channel complexity due to reduced woody debris inputs.
Certain human activities and land uses can directly introduce ammonia into aquatic systems, and in many cases ammonia-related impairments are only seen when these direct discharges are present. Sources associated with agriculture include animal wastes from concentrated animal feeding operations (CAFOs), other livestock operations, and aquaculture facilities, as well as fertilizers applied to cropfields. Sources associated with urban and suburban development include fertilizers applied to golf courses and lawns; human wastes from sewer and septic systems and wastewater treatment plants; landfill wastes; and nitrogenous vehicle emissions.
Industries (e.g., coal-fired power plants and other industrial facilities) also may release ammonia to the environment. Ammonia from these sources can be introduced into aquatic systems via four main transport pathways (or transport-defined sources): stormwater runoff, leakage or leachate into groundwater sources, atmospheric emissions and deposition, or direct effluent discharges. Each of these transport-defined sources can lead to increased ammonia inputs into surface waters.
In streams, ammonia may be dissolved in the water column or associated with sediments. At non-toxic concentrations, ammonia acts as a nutrient and can stimulate microbial and plant production (see the nutrient module for more information on these pathways). Ammonia concentrations also will depend upon the nitrogen cycle, or the transformation of nitrogen among different oxidation states. This cycle is dependent on microbial activity and dissolved oxygen levels, so these factors play an important role in determining ammonia concentrations.
At high enough concentrations, ammonia can be toxic to aquatic organisms. In general, unionized ammonia (NH3) is the form most toxic to aquatic biota. The relative contribution of unionized versus ionized forms to total ammonia concentrations depends on certain water quality criteria, most notably pH: as pH increases, so does the proportion of ammonia in its unionized form. Stressors such as ionic strength and temperature also may influence ammonia toxicity, through their effects on ammonia tolerance and toxicity.
Linking Proximate Stressors to Biological Responses
When ammonia concentrations or fluctuations increase within streams, ammonia concentrations within aquatic organisms may rise owing to reduced nitrogen excretion and increased diffusion of ammonia across gill membranes. Increases in internal ammonia concentrations can have several detrimental effects, including reduced immune, osmoregulatory, nervous system and respiratory function. For example, Na+ efflux rates are elevated in the presence of ammonia, and fish must increase Na+ influx to remain in Na+ balance.
These different modes of action all may contribute to decreased condition, decreased growth, altered behavior and increased susceptibility to other stressors in affected biota. For example, increased ammonia concentrations may result in increased gill and organ damage, increased fin erosion, or increased mucous secretion in fish; possible changes in behavior include hyperexcitability, hyperventilation and convulsions.
Ultimately, these effects may result in changes in population and community structure and ecosystem function. For example, taxa particularly sensitive to unionized ammonia, such as unionid mussels and fish, may decrease, while more tolerant organisms become more dominant.
Literature Reviews
This section presents an annotated bibliography of references providing information on stressor-response relationships for ammonia, as well as general background information. This is not meant to be a comprehensive bibliography of references dealing with ammonia, but rather is meant to highlight a few references that may be especially useful.
- Camargo J, Alonso A (2006) Ecological and toxicological effects of inorganic nitrogen pollution in aquatic ecosystems: a global assessment. Environment International 32:831-849.
This paper discusses three major effects of inorganic nitrogen pollution, in the form of NH4+, NH3, NO2-, HNO2, and NO3-, in detail: acidification of freshwater ecosystems without a large acid-neutralizing capacity, proliferation of primary producers causing eutrophication, and toxicity to aquatic vertebrates and invertebrates. The paper also describes human health and economic risks of inorganic nitrogen pollution.
- Camargo J, Alonso A (2007) Inorganic nitrogen pollution in aquatic ecosystems: causes and consequences. The Encyclopedia of Earth Web site.
This document discusses the effects of the increasing inorganic nitrogen pollution. The document explains about acidification of freshwater ecosystems, eutrophication, toxic algae, toxicity of inorganic nitrogen, in the form of NH4+, NH3, NO2-, and NO3-, to aquatic organisms and its adverse effects on human health.
- Clement B, Merlin G (1995) The contribution of ammonia and alkalinity to landfill leachate toxicity to duckweed. Science of the Total Environment 170:71-79.
This study uses toxicity testing to determine if ammonia and alkalinity are major factors in the toxicity of landfill leachate to duckweed. Landfill leachate samples are collected and used in the toxicity tests with different pH values. In addition, some leachate samples were stripped of ammonia and used to help identify effects due to ammonia.
- Constable M, Charlton M, Jensen F, McDonald K, Craig G, Taylor K (2003) An ecological risk assessment of ammonia in the aquatic environment. Human and Ecological Risk Assessment 9(2):527-548.
This paper is a case study that investigates the potential for lethal and sub-lethal effects to a mix of vertebrate and invertebrate species in freshwater bodies in Canada. The Red River and Hamilton Harbor both have numerous municipal wastewater treatment plants discharging into them, and have shown high levels of ammonia in the past. The paper includes several aquatic community risk models that were designed to show species sensitivities to ammonia.
- Diamond J, Mackler D, Rasnake W, Gruber D (1993) Derivation of site-specific ammonia criteria for an effluent-dominated headwater stream. Environmental Toxicology and Chemistry 12:649-658.
This paper looks at the range of sensitivities to ammonia for several vertebrate and invertebrate species that inhabit the eastern shore of the United States. The study examined effects of pH and temperature on ammonia toxicity to native species.
- Environment Canada and Health Canada (2001) Priority Substances List Assessment Report for Ammonia in the Aquatic Environment. Minister of Public Works and Government Services, Canada. [Archived]
This document thoroughly discusses the basic properties, sources, and effects of ammonia to the aquatic environment of Canada.
- IPCS (International Programme on Chemical Safety) (1986) Environmental Health Criteria 54: Ammonia. United National Environment Programme, International Labour Organisation, World Health Organization.
This document gives an overall detailed review of ammonia. Its topics range from chemical properties to environmental and human exposure effects.
- Scott D, Lucas M, Wilson R (2005) The effect of high pH on ion balance, nitrogen excretion and behavior in freshwater fish from a eutrophic lake: a laboratory and field study. Aquatic Toxicology 73:31-43.
This paper focuses on the effect of summer blooms of cyanobacteria and increasing pH in the Slapton Ley, a freshwater hyper-eutrophic lake, to nonsalmonid species of fish. The summer blooms of cyanobacteria and increase in pH were said to be associated with intensification of effects from agriculture and wastewater treatment plants, in part due to NH3.
- Stumm W, Morgan JJ (1996) Aquatic Chemistry. Wiley-Interscience, New York NY.
This standard text in limnochemistry provides a good explanation of the chemistry of ammonia in surface waters.
- U.S. EPA (1999) 1999 Update of Ambient Water Quality Criteria for Ammonia. U.S. Environmental Protection Agency, Washington DC. EPA-822-R-99-014. 153 pp.
This document provides an update to the 1998 update of the ambient water quality criteria for ammonia. It reviews acute and chronic toxicity of ammonia to freshwater organisms.
- Webster J, Mulholland P, Tank J, Valett M, Dodds W, Peterson B, Bowden W, Dahm C, Findlay S, Gregory S, Grimm N, Hamilton S, Johnson S, Marti E, McDowell W, Meyer J, Morrall D, Thomas S, Wollheim W (2003) Factors affecting ammonium uptake in streams: an inter-biome perspective. Freshwater Biology 48:1329-1352.
This paper discusses the Lotic Intersite Nitrogen eXperiment (LINX), which was an effort to find out how different physical, chemical, and biological process in streams affect ammonium uptake. The study looked at 11 stream sites, ranging from Alaska to Puerto Rico. At each site ammonium was introduced at the upstream portion of the study reach. Timed samples were then collected at the downstream portion of the reach, and the data from all 11 regions were compared.
- Webster J, Swank W (1985) Within-stream factors affecting nutrient transport from forested and logged watersheds. in: Blackmon R (Eds). Proceedings of Forester and Water Quality: A Mid-South Symposium. University of Arkansas.
This paper describes nutrient uptake by means of autotrophic and heterotrophic processes, macroinvertebrate particle generation, and retention of dissolved and particulate nutrients by woody debris. The study focuses on the effect of logging and the resulting change in physical stream characteristics on nutrient input and uptake.
References
- Bosakowski T, Wagner EJ (1994) A survey of trout fin erosion, water quality, and rearing conditions at state fish hatcheries in Utah. Journal of the World Aquaculture Society 25(2):308-316.
- Camargo J, Alonso A (2006) Ecological and toxicological effects of inorganic nitrogen pollution in aquatic ecosystems: a global assessment. Environment International 32:831-849.
- Camargo J, Alonso A (2007) Inorganic nitrogen pollution in aquatic ecosystems: causes and consequences. The Encyclopedia of Earth Web site.
- Clement B, Merlin G (1995) The contribution of ammonia and alkalinity to landfill leachate toxicity to duckweed. Science of the Total Environment 170:71-79.
- Constable M, Charlton M, Jensen F, McDonald K, Craig G, Taylor K (2003) An ecological risk assessment of ammonia in the aquatic environment. Human and Ecological Risk Assessment 9(2):527-548.
- Diamond J, Mackler D, Rasnake W, Gruber D (1993) Derivation of site-specific ammonia criteria for an effluent-dominated headwater stream. Environmental Toxicology and Chemistry 12:649-658.
- Eaton AD, Clesceri LS, Rice EW, Greenberg AE, Franson MAH (Eds.) (2005) Standard Methods for the Examination of Water and Wastewater (21st edition). American Public Health Association, Washington DC.
- Environment Canada and Health Canada (2001) Priority Substances List Assessment Report for Ammonia in the Aquatic Environment. Minister of Public Works and Government Services, Canada.
- IPCS (International Programme on Chemical Safety) (1986) Environmental Health Criteria 54: Ammonia. United National Environment Programme, International Labour Organisation, World Health Organization.
- Lang T, Peters G, Hoffman R, Meyer E (1987) Experimental investigations on the toxicity of ammonia: effects on ventilation frequency, growth, epidermal mucous cells, and gill structure of rainbow trout Salmo gairdneri. Diseases of Aquatic Organisms 3:159-167.
- Lapota D, Duckworth D, Ward J (2000) Confounding Factors in Sediment Toxicology - Issue Papers 1-19. Space and Naval Warfare Systems Center, San Diego CA.
- Lease H, Hansen J, Bergman H, Meyer J (2003) Structural changes in gills of Lost River suckers exposed to elevated pH and ammonia concentrations. Comparative Biochemistry and Physiology, Part C 134:491-500.
- Mancl K, Veenhuizen M (1991) Avoiding Stream Pollution from Animal Manure. Ohio State University Extension. AEX-708-91.
- Milne I, Seager J, Mallett M, Sims I (2000) Effects of short-term pulsed ammonia exposure on fish. Environmental Toxicology and Chemistry 19(12):2929-2936.
- Newton N, Bartsch M (2006) Lethal and sublethal effects of ammonia to juvenile Lampsilis mussels (Unionidae) in sediment and water-only exposures. Environmental Toxicology and Chemistry 26:2057-2065.
- NOAA (2000) Atmospheric Ammonia: Sources and Fate. A Review of Ongoing Federal Research and Future Needs (PDF). Committee on the Environment and Natural Resources, Air Quality Research Subcommittee. 19 pp.
- Sampio L, Wasielesky W, Miranda-Filho K (2002) Effect of salinity on acute toxicity of ammonia and nitrate to juvenile Mugil platanus. Bulletin of Environmental Contamination and Toxicology 68:668-674.
- Scott D, Lucas M, Wilson R (2005) The effect of high pH on ion balance, nitrogen excretion and behavior in freshwater fish from a eutrophic lake: a laboratory and field study. Aquatic Toxicology 73:31-43.
- Stumm W, Morgan JJ (1996) Aquatic Chemistry. Wiley-Interscience, New York NY.
- Twitchen I, Eddy F (1994) Sublethal effects of ammonia to freshwater fish. in: Muller R, Lloyd R (Eds). Sublethal and Chronic Effects of Pollutants on Freshwater Fish. FAO and Fishing News Books, Oxford UK.
- U.S. EPA (1999) 1999 Update of Ambient Water Quality Criteria for Ammonia. U.S. Environmental Protection Agency, Washington DC. EPA-822-R-99-014. 153 pp.
- Webster J, Mulholland P, Tank J, Valett M, Dodds W, Peterson B, Bowden W, Dahm C, Findlay S, Gregory S, Grimm N, Hamilton S, Johnson S, Marti E, McDowell W, Meyer J, Morrall D, Thomas S, Wollheim W (2003) Factors affecting ammonium uptake in streams: an inter-biome perspective. Freshwater Biology 48:1329-1352.
- Webster J, Swank W (1985) Within-stream factors affecting nutrient transport from forested and logged watersheds. in: Blackmon R (Eds). Proceedings of Forester and Water Quality: A Mid-South Symposium. University of Arkansas.
- Wilson J, Iwata K, Iwama G, Randall D (1998) Inhibition of ammonia excretion and production in rainbow trout during severe alkaline exposure. Comparative Biochemistry and Physiology, Part B 121:99-109.
- Wurts W (2003) Daily pH cycle and ammonia toxicity. World Aquaculture 34(2):20-21.
Contacts: Authors & Contributors